by Ryan Walton, J.O. Miller, Lance Champagne
As presented at the 2019 Winter Simulation Conference
Abstract
The crops of wheat, maize, and rice make up almost two-thirds of the world’s dietary needs. Additionally, soybeans account for three-quarters of global livestock feed. Considering over half of the world’s exported supply of these commodities is exported via maritime means, the free flow of marine traffic becomes paramount. Current optimization models found in a number of studies involving food commodities lack the ability to capture the inherent variance in maritime transport. To capture this variance, a discrete-event simulation was built to understand how disruptions in this system impact those who rely on its unhindered functionality. Monthly export data are used, and the maritime chokepoints of the Panama Canal, the Suez Canal, and the Strait of Gibraltar are modeled for disruption. Results indicate significant food shortages for all importers studied. Marine traffic through the Strait of Malacca was also significantly impacted when any of the three chokepoints studied were closed.
Introduction
With globalization and the global economy, the world is now more connected than ever. Never has there been an age where nations depended so heavily on other nations for resources (i.e., oil). Merriam-Webster Online (2019) defines a resource as “a natural feature or phenomenon that enhances the quality of human life.” Oil certainly makes life easier, but it is only there to “enhance.” What then, if not oil, would be a resource that is a requirement for human life? One answer is food, which can be captured in a model using a representative set of cereals and soybeans (Jones and Ejeta 2016; Wellesley et al. 2017).
Maize, rice, and wheat make up almost two-thirds of the world’s dietary energy needs (Jones and Ejeta 2016). In 2017, just six countries (United States, Russia, Argentina, Ukraine, Canada, and Brazil – in descending order of trade value) provided 55% of the global supply. Furthermore, soybeans account for three-quarters of global livestock feed, and only three countries (Brazil, United States, and Argentina – in descending order of trade value) provided 86% of the global supply (Wellesley et al. 2017; United Nations Statistics Division 2019). These megacrops are the backbone of the global food supply, and only a handful of countries export them. According to Bailey and Wellesley (2017), 2.8 billion people are fed via food imports from the global transport system each year. Assuming a population of seven billion people in the world, this equates to 40% of the world’s population being reliant on global transport to meet their daily caloric needs. Major importers of these food commodities are China, Japan, Egypt, and Spain.
Background
Maritime Transportation System
Given the global requirement for imported food, the free flow of transportation becomes important. A large proportion of the world’s traded cereals and soybeans are shipped via ocean and sea (Bailey and Wellesley 2017). Once loaded, these ships follow accepted maritime shipping routes, as shown in Figure 1.

Figure 1: Eurasia maritime shipping routes.
As can be seen in Figure 1, ship traffic concentrates around global maritime chokepoints. A chokepoint is “a strategic narrow route providing passage through or to another region” (Merriam-Webster Online 2019). In the case of maritime shipping, these chokepoints connect one geographic body of water to another. The disruption of the free flow of global cereals and soybeans through these chokepoints is the focus of this study.
Maritime chokepoint analysis on oil is prevalent (U.S. Energy Information Administration 2014; Komiss and Huntzinger 2011). However, since not all oil exporting countries are food exporting countries, the importance of food chokepoints might differ from existing oil chokepoints, or introduce new chokepoints entirely. An estimated 55% of globally-traded cereals and soybeans are shipped via maritime means that pass through at least one maritime chokepoint (Bailey and Wellesley 2017). Unlike oil, chokepoint analysis for the global food supply is scant.
Maritime Shipping Simulations
Given the global use of maritime shipping and its importance to economic well–being, the modeling of maritime networks is a frequent endeavor. Ducruet (2016) modeled global maritime flows as complex networks to measure the vulnerability of global maritime trade flows through the Suez and Panama Canals. Viljoen and Joubert (2016) also used complex network theory to model global container shipping, systematically removing links and nodes to determine the global maritime network’s robustness and flexibility. These models used optimization techniques, minimizing either cost or distance. However, if a supply chain displays variance (demand variance, quality variance, supplier variance, etc.), then methods of optimization are inadequate and simulation is then the tool of choice (Ingalls 1998). Furthermore, given the size and complexities of supply chain networks, simulation is considered a valid approach since it can incorporate uncertainties and adverse external events (Deleris and Erhun 2005).
Specifically, concerning the variation introduced by weather, ship speeds, and varying crop yields, simulation has been the preferred analytic method of choice to capture this variability. Qu and Meng (2012) used a Cellular Automata (CA) simulation model in conjunction with a discrete-event simulation to simulate ship movements through the Singapore Strait. Caris et al. (2011) developed a discrete-event simulation to model alternative transport options for container barges in the port area of Antwerp. Smith et al. (2009) modeled ship congestion in the upper Mississippi River with a discrete-event simulation, examining ship activities under a wide range of operating conditions.
Concerning global chokepoints, K¨ose et al. (2003) used a discrete-event simulation to model ship traffic flowing through the ˙Istanbul Strait, finding that an increase of ship arrivals of just 36% causes ship waiting times to jump from a mere 16 minutes to 918 minutes. Mavrakis and Kontinakis (2008) built a similar discrete-event simulation to model maritime traffic through the Strait as well and arrived at similar results. Lopes et al. (2017) constructed a discrete-event simulation to improve the efficiency of Brazil’s soybean exports.
A notable contribution to the study of maritime chokepoints in the global food supply has been by Wellesley et al. (2017) and Bailey and Wellesley (2017). The authors reconciled multiple maritime shipping databases into one single database. This single database was then used to power the Chatham House Maritime Analysis Tool (CH-MAT), an Excel-based tool that models global imports and exports. The tool then allowed the authors to identify which chokepoints were most important to certain cereals, and then determine which countries were most at risk to chokepoint disruptions. However, no stochastic elements or seasonal trends were considered.
Methodology
Model Development
focus on chokepoint disruption. The model was built in Simio and follows the transport methodology set fourth by Woxenius (2007). Ships are modeled as entities and carry a 55,000 dry weight tonnage (Handymax/Supramax class). Each month, metric tonnes of wheat, maize, and soybeans are shipped dry bulk to their destination. Ships travel directly to their destinations, as transshipping or refueling is not considered.
Distance is measured using the ArcGIS World Imagery functionality in Simio, incorporating a hiresolution scale satellite map in the background. This functionality also permits placing model objects using lat/long coordinates (way-points), which allows for accurate distance measurements.
The importing countries of China, Japan, Egypt, and Spain are selected due to their large import reliance of at least one of the commodities under study. Given these four importers, six common exporters are selected - the United States, Brazil, Canada, Argentina, Ukraine, and Russia. Using these six exporters provides a high percentage of an importer’s annual supply of a commodity. Travel speed is stochastic and is modeled via a triangular distribution, with maximum and minimum values within 10% of the mean travel speed of 14 mph. Monthly commodities data is also stochastic via a triangular distribution with maximum and minimum values within 10% of the United Nations’ reported monthly export amount for a specific exporter. A triangular distribution is used to provide a reasonable amount of variation for these input metrics since only a single value was available for each.
Ship entities originate at an exporting country’s nearby port. Specifically, the exporting countries of Brazil, Canada, and the United States all have two exporting locations - either East/West or North/South. The United States exports near New Orleans (East) and Seattle (West). Canada exports near Newfoundland (East) and Vancouver (West). Brazil exports near S˜ao Luis (North) and S˜ao Paulo (South). All other exporters are single-origin, with Argentina exporting near Buenos Aries, Ukraine near Odessa, and Russia near Novorossiysk. All importers are single-destination, with China importing near Wenzhou, Japan near Tokyo, Egypt near Alexandria, and Spain near Valencia.
Initial route selections are determined via distances collected from MarineTraffic (2019) and are modeled herein. However, during a chokepoint disruption, a ship will reroute itself using whichever route is shortest in distance from its current location. This occurs dynamically, as the ship entity is continuously aware of which alternate route is fastest should a chokepoint close. The same process is used when a chokepoint reopens, as the reopened route might now be shortest.
Supporting Data, Verification, and Validation
Commodities data used in this model are sourced from the United Nations International Trade Statistics Database. This data is available in both monthly and annual time-frames, and is available publicly (United Nations Statistics Division 2019).
Monthly exports of wheat, maize, and soybeans from United Nations Statistics Division (2019) are used to determine demand. Monthly data are preferred to annual because the commodities under study are seasonal - the amount of grain a country exports depends on the harvest season/month. Using monthly data provides insight not only into which chokepoints are most critical for the shipment of these foodstuffs, but also how the impact varies with the disruption month.
Both TXR Logistics (2018) and the MarineTraffic (2019) database are used to validate model routes and distances. TXR Logistics is a global freight-forwarder based in the United States, specializing in global air and maritime import and export for domestic and international clients. MarineTraffic is a leader in ship tracking, using historical Automatic Identification System (AIS) data to provide accurate estimates of ship movements. The AIS is a vessel tracking system that utilizes a ship’s on-board transponder to track its whereabouts. Using MarineTraffic’s Voyage Planner, the distance of 37 unique simulated routes are compared to their matching real-world routes. Of the 348,154 cumulative miles the 37 routes cover, the model is within 0.0012%, or 4.17 miles.
Results
Overview
The aforementioned four importing countries and six exporting countries are considered for the disruption analysis, with the maritime chokepoints of the Panama Canal, the Suez Canal, and the Strait of Gibraltar being individually closed to all ship traffic. Chokepoints are closed for 30 days, then reopened. Monthly exports of wheat, maize, and soybeans are considered.
Four separate scenarios are analyzed: a baseline scenario with all chokepoints open, and three additional scenarios each with a single chokepoint being closed. Each scenario has a three-month warm-up, then continues for an additional four, resulting in a seven-month run length. In scenarios where a chokepoint is closed, the closure occurs in the middle of month four, then is reopened 30 days later in the middle of month five. The simulation continues to run until the conclusion of month seven, providing 2.5 additional months for the system to recover from the disruption.
Closure scenarios have a closure occurring in each of the 12 months in 2017. However, the historical data required is from 2016-2018. This wide range is due to how the simulation is structured. Given that each month in 2017 has four unique scenarios tested (one baseline with three different closures), each month therefore requires a different set of historical data for a total of 12 different sets. For example, scenarios with a chokepoint closed in January 2017 require the three months preceding January 2017 (the warm-up period) and the four months thereafter. Therefore, to analyze the impact of closures in this specific month, a data set beginning on 01 October 2016 and ending on 30 April 2017 is required. Likewise, scenarios with a December 2017 closure require a 01 September 2017 - 31 March 2018 set of historical data. All scenarios, including the baseline, use this methodology.
All scenarios are run for 30 replications and a statistical difference in average monthly imports for a commodity between the baseline (no disruption) and a disruption scenario (one of the three chokepoints) is determined at a .05 alpha level. These differences are calculated for each of the three months following the disruption and reported as a percentage change of the average monthly imports. Bold values found in tables presented in the following sections indicate statistically significant changes in average imports for one of the months (typically month five immediately following the disruption) for the given disruption month. For cases where more than one month following the disruption showed a statistically significant change, the maximum monthly difference is reported and is denoted by an asterisk (*) in the table.
The Panama Canal
The Panama Canal runs through Panama and connects the Atlantic and Pacific Oceans, providing a shorter maritime route for east/west shipping.
Closing the Panama Canal for 30 days revealed a statistically significant decrease in monthly wheat imports to Japan and Egypt, as shown in Table 1. Japanwas most sensitive, experiencing significant decreases in nine of twelve disruption months. Egypt was less dependent, experiencing a significant decrease in only one disruption month. Egypt’s resilience in wheat imports is likely due to its sourcing over 80% of its imported wheat from Russia and Ukraine (United Nations Statistics Division 2019). Neither exporter utilizes the Panama Canal to reach Egypt. However, both Russia and Ukraine must use the Turkish Straits for maritime shipping, which is one of the narrowest chokepoints in the world and has no alternate maritime route. The Turkish Straits are not considered in this analysis. Wheat imports to Spain are under-represented by this research and are therefore omitted (denoted by “-”). Spain sourced only 10% of its 2017 wheat imports from the six exporters in this study (United Nations Statistics Division 2019).
Table 1: Wheat via Panama Canal.
Table 2 shows that soybean imports were significantly impacted when the Panama Canal closed. According to United Nations Statistics Division (2019), China, Japan, and Spain rely heavily on North and South America for their soybean imports. China is most reliant on the United States and Brazil, with both countries supplying 89% of total soybean exports to China in 2017. China’s sensitivity from closing the Panama Canal occurs when importing soybeans from the United States, which utilizes the Panama Canal to access the Pacific Ocean (these months are also common soybean harvest months in the United States). Brazil bypasses the Panama Canal, as the southern route utilizing the Cape of Good Hope and the Strait of Malacca is shorter. Japan, however, is most reliant on the United States and Canada for their soybean imports, receiving 84% of total imports from these two exporters in 2017. Both countries utilize the Panama Canal to reach the Pacific. Even though Spain is east of the Panama Canal, shipments from the west coast of the United States and Canada still traverse the Panama Canal to reach Spain. Egypt experienced no significant impact from a Panama Canal closure, as it sourced almost 50% of its 2017 soybean imports from exporters that bypass the Panama Canal (United Nations Statistics Division 2019).
Table 2: Soybeans via Panama Canal.
Maize shipments between the six exporters and the four importers revealed no significant decreases in monthly shipments. According to the United Nations Statistics Division (2019), China, Japan, Egypt, and Spain sourced their 2017 maize imports from countries that either bypass the Panama Canal completely, or use it only for a portion of the total shipment.
The Strait of Gibraltar
The Strait of Gibraltar connects the Atlantic Ocean with the Mediterranean Sea, and is part of a sea route connecting the West to the East.
Wheat imports were impacted in only two of twelve disruption months for both China and Egypt, as displayed in Table 3. The impact to China can be traced back to its wheat imports from eastern Canada, as these shipments traverse the Strait of Gibraltar. Egypt is also sensitive to a closure here, most likely due to it importing wheat from the United States and Argentina, both of which use the strait as well.
Table 3: Wheat via Strait of Gibraltar.
Maize imports given a Strait of Gibraltar closure highlight an Egypt and Spain dependency on maize from western exporters, as detailed in Table 4.
Egypt and Spain imported 34% and 27% of their maize, respectively, from Ukraine (United Nations Statistics Division 2019). When shipping to these importers, Ukraine does not utilize the Strait of Gibraltar. All other maize exporters represented in this research, however, utilize the Strait of Gibraltar and would have to subsequently reroute around the Cape of Good Hope, then traverse Bab-el-Mandeb and the Suez Canal to reach Egypt and Spain, a voyage that would add weeks to any shipment and add additional cargo risk as ships traverse additional chokepoints.
Table 4: Maize via Strait of Gibraltar.
Soybeans are heavily sourced from western countries, and much like maize, this commodity must also utilize the Strait of Gibraltar to reach Egypt and Spain. Table 5 contains the impact a closure of the Strait of Gibraltar has on soybean imports.
Table 5: Soybeans via Strait of Gibraltar.
According to the United Nations Statistics Division (2019), both Egypt and Spain sourced most of their soybean imports in 2017 from the United States, Brazil, and Argentina - all countries that utilize the Strait of Gibraltar to reach these importers. While both countries would see significant monthly decreases in their soybean imports, Egypt is still better positioned as it received 23% of its 2017 soybean imports from Ukraine, bypassing the Strait of Gibraltar all together.
The Suez Canal
The Suez Canal runs through Egypt and connects the Mediterranean Sea to the Red Sea. Much like the Strait of Gibraltar, the Suez Canal connects West to East.
Closing the Suez Canal had a significant impact on Chinese wheat imports (Table 6), with six of twelve disruption months displaying a significant decrease.
Table 6: Wheat via The Suez Canal.
China sourced 15% of its 2017 wheat imports from Canada, and when exported from Canada’s east coast these exports traverse the Suez Canal. Closing the Suez Canal forces ships to turn around and exit the Mediterranean through the Strait of Gibraltar, adding up to a week of additional travel time.
Chinese maize imports appear to be impacted as well if the Suez Canal closes, as depicted in Table 7. According to the United Nations Statistics Division (2019), Ukraine supplied China with over 61% of its maize imports in 2017, and utilizes the Suez Canal to ship east to China. China also sourced 27% of its 2017 maize imports from the United States - an exporter that bypasses the Suez Canal in favor of the Panama Canal when shipping to China. This provides China a buffer should the Suez Canal close.
Table 7: Maize via The Suez Canal.
Soybean shipments with the Suez Canal closed resulted in no significant decreases in the four importing countries studied. This is likely due to where these four importers reside geographically relative to the exporters chosen to study. Soybeans exported to China and Japan utilize the Panama Canal, and those exported to Egypt and Spain utilize the Panama Canal, the Strait of Gibraltar, and the Turkish Straits (those exported from Ukraine).
Impact of Closures on the Strait of Malacca
According to the U.S. Energy Information Administration (2014), the Strait of Malacca is one of the world’s most important strategic chokepoints by volume of oil transit, connecting the Indian Ocean to the Pacific Ocean. In 2016, oil flows through here rose to 16 million barrels per day, and it is considered the second busiest transit chokepoint behind the Strait of Hormuz. Oil shipments through the Strait of Malacca supply the growing economies of China and Indonesia.
Given the Strait of Malacca’s importance in global trade, the free flow of ship traffic becomes paramount. However, this free flow could be jeopardized with the closure of a chokepoint half a world away. When a chokepoint closes, ships will reroute to their destination using the shortest route available. If a ship is destined for China and the Panama Canal closes, the shortest route often available is one utilizing the Strait of Malacca. Should an entire fleet of exports need to reroute to traverse the Strait of Malacca and not be able to pass due to increased arrivals, the free flow of ship traffic is no longer viable and the system could come to an abrupt halt.
Figure 2 depicts monthly expected arrival increases to the Strait of Malacca should the Panama Canal close for 30 days during the listed month (using 2017 data). To read Figure 2, the listed values are offset by two months (due to the large distance between the Panama Canal and the Strait of Malacca). For example, if the Panama Canal closed in January, the listed increase of 80.0% occurs two months later in March.
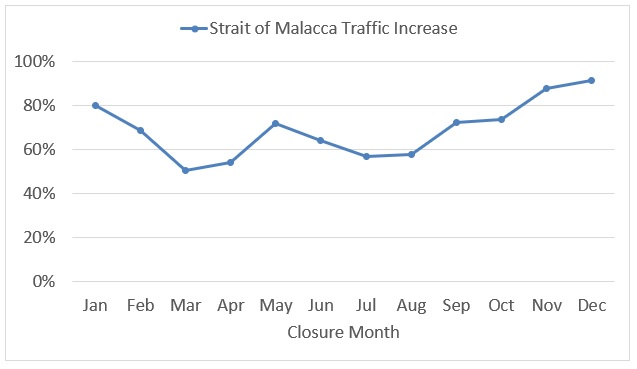
Figure 2: Panama Canal closure.
Table 8 contains all traffic increases/decreases to the Strait of Malacca, with the column values for the Strait of Gibraltar and Suez Canal also being interpreted with an offset. However, these values are offset by only one month.
According to Table 8, should the Panama Canal close, the Strait of Malacca could expect at least a 51% arrivals increase (in the exports studied) in all months, as compared to the baseline, with a maximum increase of nearly double if the Panama Canal closed in the month of December.
Should the Strait of Gibraltar close, arrival increases of at least 20% could be expected in all months, with the largest impact coming from a closure in January. This is likely due to exports emanating from the western United States and Canada to Egypt and Spain, as the primary route is to utilize the Panama Canal then the Strait of Gibraltar. The alternate route for these exporters is to then utilize the Strait of Malacca.
A closure of the Suez Canal, however, results in traffic decreases in the Strait of Malacca. These decreases are likely attributed to ship traffic originating in Russia, Ukraine, and Eastern Canada with a destination of Japan. With an eastward heading, these exporters utilize the Suez Canal and eventually the Strait of Malacca to export to Japan. Should the Suez Canal close, these exporters would traverse the Panama Canal to reach Japan, completely bypassing the Strait of Malacca.
Table 8: Strait of Malacca Traffic Increases/Decreases.
Conclusion
North and South America are top exporters of the global food supply. Given most of this food supply travels via maritime means, this exposes any importing country to substantial chokepoint disruption risk. Should a disruption happen, importers could be facing heavy shortages of diet staples, which could potentially result in famine if protective measures are not taken. Shipping costs could skyrocket, as shipping companies are accumulating more distance to reroute around a closure. A shipping cost increase would likely be passed on to the consumer, which could cause the market for these commodities to also skyrocket.
Should the Strait of Malacca experience the increased traffic as detailed in this analysis, oil tankers traversing there will be slowed if not stopped. If oil tankers, or any other valuable commodity, are slowed or stopped in the Strait of Malacca, cargo safety will be a concern as the waters there have a history of piracy (Hassan and Hasan 2017).
Given the increasing size of the global economy, the free flow of goods becomes more important each day. Although the event of any one chokepoint closing is extremely rare, the consequences can be painfully high.
Acknowledgments
The views expressed in this article are those of the authors and do not reflect the official policy of the United States Air Force, Department of Defense, or the US Government.
Author Biographies
RYAN WALTON earned a B.A. in Economics (2009) from New Mexico State University and his M.S. in Operations Research (2019) from the Air Force Institute of Technology (AFIT). He currently serves as a Captain in the United States Air Force and is stationed at Wright-Patterson Air Force Base, Ohio as an operations research analyst for Air Force Materiel Command. His research interests include computer simulation and operations research with application in geopolitics, demographics, and strategy. His email address is ryan.walton.2@us.af.mil.
J. O. MILLER is a 1980 graduate of the U.S. Air Force Academy (USAFA) and retired from the Air Force as a Lt. Colonel in January 2003. In addition to his undergraduate degree from USAFA, he received an MBA from the University of Missouri at Columbia in 1983, his M.S. in Operations Research from the Air Force Institute of Technology (AFIT) in 1987, and his Ph.D. in Industrial Engineering from The Ohio State University in 1997. He is an Associate Professor of Operations Research in the Department of Operational Sciences at AFIT. His research interests include combat modeling, computer simulation, and ranking and selection. His email address is ohn.miller@afit.edu.
Engineering from Tulane University (1991), M.S. in Operations Research (1999) and Ph.D. in Operations Research (2004) from the Air Force Institute of Technology (AFIT). He is an Assistant Professor of Operations Research in the Department of Operational Sciences at AFIT. His research interests include agent-based simulation, combat modeling, and multivariate analysis techniques. His email address is lance.champagne@afit.edu.